Kazuhiko Seki (Leader), Nanoscale Theory Group, the Nanosystem Research Institute (Director: Tomohiko Yamaguchi) of the National Institute of Advanced Industrial Science and Technology (AIST; President: Ryoji Chubachi), Akihiro Furube (Senior Researcher), Nanoscale MicroSpectroscopic Analysis Group, the Research Institute of Instrumentation Frontier (Director: Yukihiko Yamauchi) of AIST, and Yuji Yoshida (Leader), Advanced Low Cost Processing Team, the Research Center for Photovoltaic Technologies (Director: Shigeru Niki) of AIST, calculated the theoretical limit of the photoelectric conversion efficiency of organic solar cells, which have gained attention as a new generation of solar cells.
The theoretical limit of the photoelectric conversion efficiency has been known for inorganic solar cells. By modifying the theory for inorganic solar cells to take into account the differences in mechanisms for producing charges after light absorption, a theoretical limit of the photoelectric conversion efficiency of organic solar cells was calculated. The results are expected to serve as guides for improving the photoelectric conversion efficiency of organic solar cells. The results will be published soon in the online version of Applied Physics Letters, a journal of the American Institute of Physics.
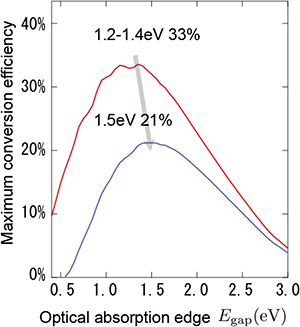 |
Relationship between the theoretical limit of the photoelectric conversion efficiency of solar cells and the minimum energy of light that can be absorbed by solar cells (optical absorption edge) when there is an energy loss of 0.4 eV during charge separation
The red line shows the theoretical limit of inorganic solar cells, and the blue line shows the new theoretical limit of organic solar cells. |
Organic solar cells are light, thin, and soft due to characteristics of organic materials. They are expected to be a new generation of solar cells capable of generating energy in locations where it was previously difficult to install solar cells. Although the materials are generally inexpensive, improving photoelectric conversion efficiency and durability has been technically difficult. However, in recent years, the photoelectric conversion efficiency has rapidly improved, and a conversion efficiency exceeding 10 % has been reported. The efficiency is as high as that of amorphous silicon solar cells. The rapid increase of the photoelectric conversion efficiency aroused interest as to how much the conversion efficiency of organic solar cells can be improved. In 1961, Shockley and Queisser showed that the theoretical limit of the photoelectric conversion efficiency for solar cells comprising inorganic semiconductors was approximately 30 %. As the actual efficiency of such solar cells is now approaching this value, recent research and development efforts for inorganic solar cells are focusing on improving efficiency through the introduction of structures such as multi-junction solar cells and concentrating solar cells that were not considered in Shockley and Queisser's theory. Meanwhile, the photoelectric conversion efficiency of organic solar cells has rapidly increased to the level where it is now desirable to calculate an efficiency limit, as Shockley and Queisser did for inorganic solar cells.
AIST researchers from various field, particularly from the Research Center for Photovoltaic Technologies, has been conducting interdisciplinary research and development to improve the efficiency and durability of organic solar cells; these researchers are from the fields of Environment and Energy, Metrology and Measurement Science, and Nanotechnology, Materials and Manufacturing. The Committee for Studying the Limit of Organic Solar Cells, initiated by Leader Yoshida and made up of AIST researchers from various fields, conducted this study on the theoretical limit of the photoelectric conversion efficiency of organic solar cells.
The photoelectric conversion efficiency of a solar cell is limited by factors such as the band gap of the semiconductor, dissipation as heat, and electric charge recombination. Light with energy less than the band gap is not absorbed and does not contribute to the generation of electricity. Light with energy higher than the band gap becomes heat and dissipates, causing a decline in voltage. If the charges that were generated by light are lost through recombination by the time it reaches the electrodes, the electric current is decreased. All of these factors decrease the electric power of the solar cell. Taking these factors into consideration, a theoretical limit of the photoelectric conversion efficiency of inorganic solar cells was shown in 1961 by Shockley and Queisser (J. Appl. Phys. vol. 32, p.510 [1961]).
The theoretical limit of the photoelectric conversion efficiency was calculated on the basis of inorganic semiconductors and was believed to be invalid for organic solar cells. In organic substances, Coulombic attraction between positive and negative charges is strong and produces bound pairs called excitons after light absorption. The Coulombic binding energy of the excitons in organic substances is estimated to be at least 10 times greater than the thermal energy at room temperature. Because the charge separation of excitons in a single organic substance is insufficient, an organic solar cell comprises two types of substances: an organic substance that tends to form positive ions and an organic substance that tends to form negative ions. At the interface between these substances, the charges of the excitons are separated. The present research focused on the presence of the excess energy required for charge separation in organic solar cells. The method of Shockley and Queisser's theory shows that when the excess energy is taken into account, the rate of charge recombination increases, resulting in voltage and current changes. Using Coulomb interaction in which 1 nm is the distance between the bound positive and negative charges and 3.5 is the general value of dielectric constant in organic substances, the excess energy required for charge separation is calculated to be 0.3 to 0.4 eV. Because there are other interactions, this value is considered to be the minimum value. Although roughly estimated, it is almost the same as the value for the minimum excess energy given in previous reports. When the theoretical limit of the photoelectric conversion efficiency was calculated using 0.4 eV as the excess energy required for charge separation, the maximum value of 21 % was obtained. The wavelength of absorbed light at which an organic solar cell demonstrates the highest efficiency has also been determined as 1.5 eV (wavelength of 827 nm) by theoretical calculations, and it provides a guide for selecting an organic molecule that absorbs light (mainly donor).
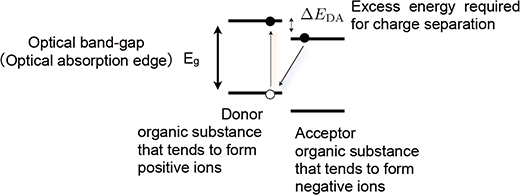 |
Figure 1 : Schematic diagram of the mechanism of charge separation in an organic solar cell
Light is usually absorbed by an organic molecule (donor) that tends to form a positive ion. An electron in the donor forms an exciton by the light absorption. Subsequently, charge separation occurs. As a result, the donor becomes a positive ion and the acceptor becomes a negative ion. In this process, the electron loses the excess energy required for charge separation (ΔEDA). |
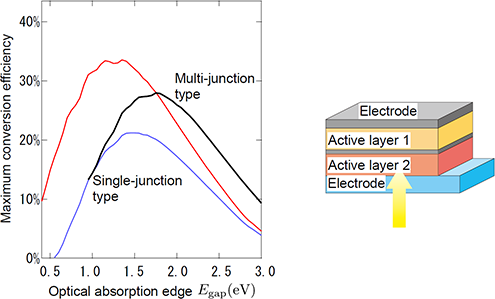 |
Figure 2 : Relationship between the theoretical limit of the photoelectric conversion efficiency and the minimum value of light energy that can be absorbed (optical absorption edge) by a multi-junction organic solar cell when the excess energy required for charge separation is 0.4 eV
It is assumed that two solar cells with a difference in optical edge energy of 0.4 eV are connected in series. The red line represents the conventional theoretical limit of single-junction inorganic solar cells, the blue line represents the theoretical limit of single-junction organic solar cells, and the black line represents the theoretical limit of multi-junction organic solar cells. |
The limit of the photoelectric conversion efficiency of single-junction organic solar cells was theoretically calculated to be 21 % using 0.4 eV as the excess energy required for charge separation. This limit value of 21 % is higher than the current efficiency of 10 % to 12 %, and it suggests that further improvement can be expected in the future as a result of material selection and structure optimization. The researchers intend to uncover the factors for the difference between the theoretical limit and actual efficiency, and expand research and development efforts to identify and solve issues for increasing efficiency.