Masaki Koike (Leader), Super-Spectroscopy System Research Group, the Research Institute of Instrumentation Frontier (Director: Masataka Ohkubo) of the National Institute of Advanced Industrial Science and Technology (AIST; President: Tamotsu Nomakuchi), has developed a superconducting nanostrip ion detector in collaboration with Roberto Cristiano, the Istituto di Cibernetica, Consiglio Nazionale delle Ricerche (CNR; Director: Russo Maurizio), and Nobuyuki Yoshikawa (Professor), Yokohama National University (President: Kunio Suzuki).
The detector with nano-structured superconducting strips, to which a bias current is applied, can detect ions through superconducting-normal transition induced by phonons (sound) produced when an ion impacts on the detector surface. A fast response time (nanosecond) comparable to that of conventional ion detectors and detection performance that does not degrade with ions of large mass are realized. This has been achieved by arranging superconducting strips (niobium or niobium nitride) that are a few tens of nanometers thick and a few hundreds of nanometers wide in series and parallel in an area of a few mm2. The nanosecond signals can be read by using a fast superconducting digital circuit. In addition, the detector can determine the charge numbers of ions by changing the bias current. The superconducting detector is expected to overcome the limits of conventional time-of-flight mass spectrometers (TOF MSs).
Details of this technology will be presented at the 60th American Society for Mass Spectrometry Conference on Mass Spectrometry and Allied Topics in Vancouver, Canada, from May 20 to 24, 2012.
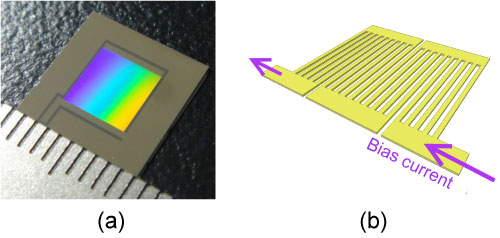 |
Photograph (a) and schematic illustration (b) of the superconducting nanostrip ion detector |
Rainbow colors are produced by diffraction by the nano structure. The scale is 1 mm (a). The niobium or niobium nitride strips
have a thickness of a few tens of nanometers and a width of a few hundreds of nanometers (b). |
TOF MSs are indispensable for the life sciences. In the spectrometer, atoms or molecules are ionized and accelerated by a voltage of a few kilovolts to fly a certain distance, and then the ions are detected by an ion detector. Measurement of the time of flight provides a way to identify the atoms or molecules or to analyze molecule structures. Ion detectors should have a fast (nanosecond) response time to ensure a sufficiently high mass resolution. In conventional TOF MS instruments, because of lower detection sensitivity of ion detectors, the measurable range is limited to a mass range less than 4000. In addition, the charge numbers of ions cannot be measured by conventional instruments, and therefore the mass of the ion cannot be determined directly.
AIST has been conducting R&D of advanced analytical instruments for science, technology, and industry. As part of its efforts, AIST has been pursuing the work to break through the technological or inherent limitations of mass spectrometry by using superconductivity technology (see AIST Today Vol. 9, No. 8).
This R&D project was supported by Grants-in-Aid for Scientific Research, Basic Research (A), "Development of a mass spectrometer equipped with a superconducting nano-stripline detector for high-mass molecules (FY2010 to FY2013)." The detector was made at AIST Nano Processing Facility using the IBEC Innovation Platform of AIST. The international collaborative research with CNR was conducted through the Postdoctoral Fellowship for Foreign Researchers of Japan Society for the Promotion of Science. In addition to ion detection, superconducting sensors can be applied to infrared photons, X-rays, and terahertz waves. The superconducting sensors are being standardized by the International Electrotechnical Commission Technical Committee 90 (IEC TC90).
Figure 1 compares the detection principles of conventional ion detectors and the developed superconducting detector. Because the energy of ions in a mass spectrometer is low (only a few keV), heavy ions such as protein ions impact against the detector surface mildly, causing adhesion to the detector surface. Conventionally, to detect collisions, one secondary electron released from the surface is amplified by electron multiplication. With increasing ion mass, secondary electron emission probability decreases, thus reducing the detection sensitivity. In the superconducting detector, on the other hand, phonon generation due to ion impact is used in order to avoid the sensitivity degradation. The phonons are created even by the mild impact, so that mass-independent detection is possible.
The superconducting detector has nano-scale strips of a superconductor (niobium or niobium nitride) with a thickness of a few tens of nanometers and a width of a few hundreds of nanometers. The nano-strips are arranged in series and parallel in an area of a few mm2. The arrangement of the nano-strips is important to realize both the excellent properties of a nanostructure and a sufficiently large sensitive area that is important for practical use. The detector becomes superconductive when cooled to about -270 °C. When the width of the strips is less than 1 µm, the phonons produced by ion impact induce a local transition from the superconducting state to the normal state, which results in formation of a normal band across the strip width. The normal band causes electric resistance. A nanosecond voltage pulse is generated by the resistance appearance, making rapid ion detection possible. Because phonons are generated by the impact of ions of any masses, ions of a wide mass range can be detected at the nanosecond time scale with high sensitivity.
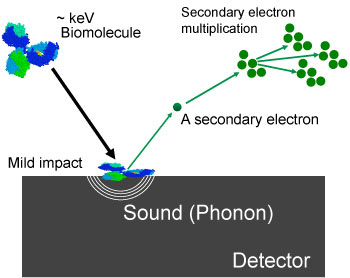 |
Figure 1 : Difference between conventional ion detection and superconducting ion detection |
In mass spectrometry, heavy ions cause only mild impact, such as adhesion to the surface of the detector.
Phonons are produced by such mild impact and the superconducting state is destroyed. |
Figure 2 shows the waveform of the voltage pulse observed when a peptide hormone ion of angiotensin I impacts on the superconducting detector. It is recognized that the pulse width is very narrow (less than 1 ns). Similarly, a nanosecond pulse was observed with immunoglobulin G multimers that had a higher molecular weight. The detector was able to detect heavy ions of a molecular weight up to 600,000.
To readout these fast pulses, a single flux quantum (SFQ) integrated circuit was developed. The SFQ circuit uses SFQs as information media. Using this circuit, mass spectra can be measured by converting the fast voltage output pulses to SFQs.
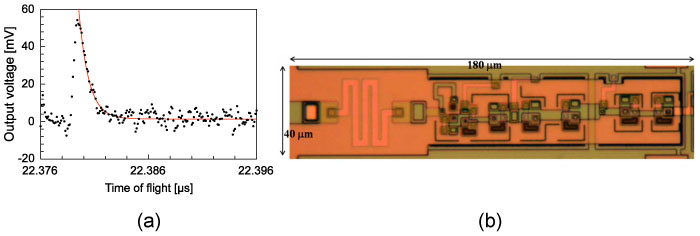 |
Figure 2 : Waveform of the nanosecond output pulse produced in response to the impact of one ion of angiotensin I (a biomolecule) (a);
and the superconducting digital circuit used to convert the output pulse to a digital signal (b) |
The SFQ circuit was fabricated by using the microfabrication process of International Superconductivity Technology Center. |
Furthermore, it has been found that the ionic charge numbers can be determined by changing the bias current applied to the detector. Figure 3 is an example of the measurement of multimer ions of an enzyme, lysozyme. When the bias current was low, as shown in Fig. 3(a), only divalent ions of monomer to hexamer were observed. When the bias current was increased, as shown in Fig. 3(b), monovalent ions of monomer to hexamer were observed mainly. Ordinary mass spectrometry cannot differentiate a monovalent ion and a divalent ion with the same m/z, for example, a monovalent monomer ion and a divalent dimer ion. However, these ions can be differentiated by using the superconducting detector. The same overlap occurs between a monovalent dimer ion and a divalent tetramer ion and so on.
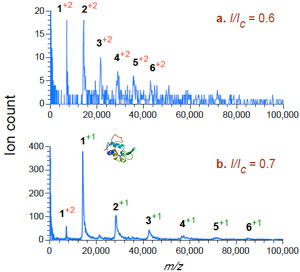 |
Figure 3 : Example of analysis of the lysozyme multimers |
For example, as the divalent dimer ion (2+2), which is produced only in small quantity, has the same
m/z as the monovalent monomer ion (1+1), it is impossible for ordinary mass spectrometers to
differentiate them. The superconducting detector can identify the weak peak of divalent dimer ions. |
To achieve diverse high-throughput analysis, the researchers plan to increase the sensitive area of the detector and improve the data-processing capacity of the superconducting digital circuit. A very low temperature of -270 °C is required to operate the developed superconducting detector. However, they have developed an automatic cooling system that does not require a supply of liquid helium. In two years, the researchers expect to have developed a time-of-flight mass spectrometer equipped with the developed superconducting detector and to be able to accept requests for analysis through the IBEC Innovation Platform of AIST.