Kazuhiro Sayama (Leader) and Rie Saitou (AIST Postdoctoral Researcher), Solar Light Energy Conversion Group, the Energy Technology Research Institute (Director: Yasuo Hasegawa) of the National Institute of Advanced Industrial Science and Technology (AIST; President: Tamotsu Nomakuchi), have developed a very high-performance multilayered photoelectrode for hydrogen production by water electrolysis using an oxide semiconductor photoelectrode. In the reaction to convert solar energy into hydrogen energy, a solar energy conversion efficiency of 1.35 % has been achieved in a carbonate electrolyte by stacking two photoelectrodes. This value is about twice the reported conversion efficiency of oxide photoelectrodes. Using solar energy, the developed technology can substantially reduce the electrolysis voltage required for hydrogen production by water electrolysis. The technology is expected to realize low-cost hydrogen production.
Details of this technology will be presented at the 7th New Energy Symposium to be held from March 13 to 15, 2012, at the University of Tsukuba (Tsukuba, Ibaraki).
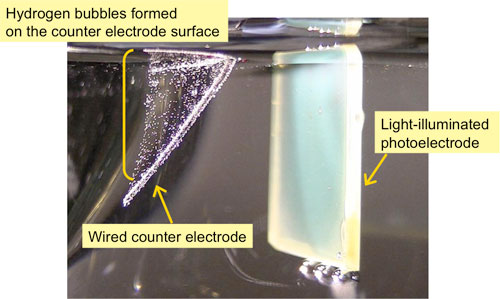 |
(Figure) : Developed high-performance photoelectrode in a carbonate electrolyte (right)
and hydrogen bubbles formed on the counter electrode surface (left)
Photoelectrode system for the conversion of solar energy into hydrogen energy |
Effective use of renewable energy is essential for reducing CO2 emissions and developing a sustainable society that does not rely on fossil resources. The use of solar energy—the most abundant renewable energy—is very important, but limited solar energy utilization technologies are available (Fig. 1). Artificial photosynthesis is the fourth solar energy utilization technology to be developed, after solar power, solar heat, and biomass technologies. Among artificial photosynthesis technologies, the solar hydrogen production technology that produces hydrogen and oxygen by directly decomposing water with a photocatalyst or a photoelectrode using an easily fabricated oxide semiconductor is a low-cost technology and has been studied extensively as a fundamental technology for the development of a future hydrogen society. The development of a solar hydrogen production system that is as highly efficient as solar cells and as simple and inexpensive as plant cultivation would contribute to the solution to the energy problems. However, reported efficiencies in converting solar energy into hydrogen energy using oxide semiconductor photoelectrodes are low (0.69 % when only an oxide is used and 1.1 % when an oxide is used in combination with expensive platinum). A high-performance system therefore needs to be developed.
AIST has been conducting research and development of hydrogen production by water electrolysis using porous photoelectrodes of various oxide semiconductors. Hydrogen production by water electrolysis using an oxide semiconductor photoelectrode is a solar energy conversion technology originated in Japan. Usually, electrolysis of water requires a voltage of more than 1.23 V theoretically but more than 1.6 V practically, owing to the effect of overvoltage. Use of a photoelectrode allows hydrogen to be produced by water electrolysis using a low-voltage auxiliary power supply (a voltage of about 0.7 V was applied to the photoelectrode in this study, but the voltage can be reduced to almost zero), thus enabling low-cost hydrogen production. In the early stages of research and development, single crystals and high-temperature sinters (pellets) of titanium oxide were used, but these materials have a drawback in that only ultraviolet light can be used. Subsequently, mostly in Europe, there has been extensive research into porous thin-film electrodes of oxide semiconductors that can use visible light (e.g. tungsten oxide (WO3) and iron oxide (Fe2O3)). The electrodes were formed on a conductive substrate by a wet process. Oxide semiconductors are mostly of the n-type and are suitable for an oxygen generating electrode. Because an oxide semiconductor film can be formed by applying the oxide semiconductor material to a substrate and sintering the material in the air, it is easy to form a large-area film. However, the efficiency of the electrode in converting solar energy into hydrogen is low and needs to be improved for practical use.
In this study, substantial improvement in the conversion efficiency by stacking three different oxide semiconductor layers to suppress charge recombination and increase light absorption.
This study was carried out in a project, “Research on Innovative Technology for Solar Energy Conversion to Chemical Energy” (FY2010– present), of Funding Program for Next Generation World-Leading Researchers by the Japan Society for the Promotion of Science.
Figure 2 shows the principle of hydrogen production by water electrolysis using an n-type semiconductor, such as titanium oxide, as a photoelectrode. The photoelectrode is connected to the counter electrode and usually an auxiliary power supply, such as a solar cell, is placed between them. When light is absorbed by the semiconductor photoelectrode, the electrons in the valence band jump into the conduction band, i.e. optical excitation. The electrons in the conduction band are transferred to the counter electrode by the auxiliary power, water is reduced on the counter electrode, and hydrogen is produced. The high energy of the electrons in the conduction band allows them to be transferred to the counter electrode by the auxiliary power supply even though its voltage is lower than the normal electrolysis voltage of water. Excitation of the electrons from the valence band leaves behind positively charged “holes.” The holes can easily remove electrons from other substances (i.e. easily oxidize other substances); oxygen is therefore produced by the oxidation of water on the photoelectrode. Thus, water can be electrolyzed at a low voltage, making it possible to build a system with reduced production cost by improving the performance of the photoelectrode, instead of producing hydrogen by electrolyzing water with only the electricity generated by solar cells (Fig. 3). If all of the light with a wavelength of up to 500 or 600 nm can be used for this reaction and the voltage of the auxiliary power supply can be reduced to almost zero, then the theoretical limit of solar energy conversion efficiency will be 8 % or 15 %, respectively. A conversion efficiency comparable to that of a system simply combining solar cells and water electrolysis would be achieved with a simple photoelectrode and fewer solar cells.
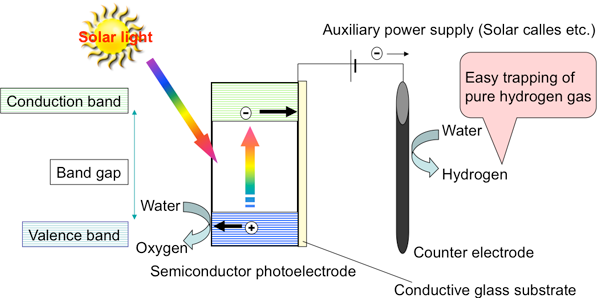 |
Figure 2 : Principle of hydrogen production by water electrolysis using a semiconductor photoelectrode |
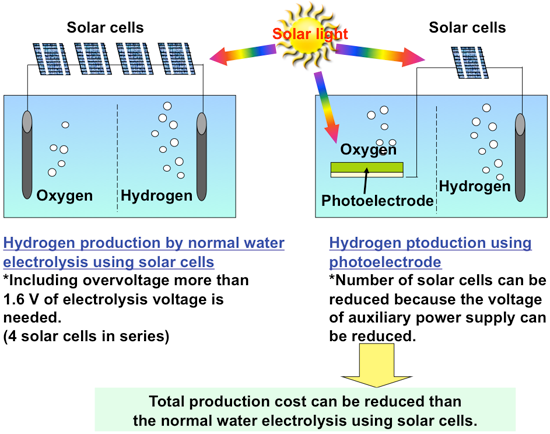 |
Figure 3 : Advantages of hydrogen production by water electrolysis using a semiconductor photoelectrode |
An oxide photoelectrode consisting of three different semiconductor layers was fabricated and hydrogen was produced by water electrolysis using a high-concentration carbonate electrolyte. Figure 4 shows a photo and electron microscope images of the multilayered photoelectrode. The photoelectrode consists of three layers of semiconductors stacked on a conductive glass substrate: tungsten oxide (WO3) as the first layer, tin oxide (SnO2) as the second layer, and bismuth vanadate (BiVO4) as the third layer. A solution containing metal ions corresponding to each layer is applied to the substrate by the spin-coating process and a thin film was formed by sintering. Porous thin-films can be formed by this method. When light is incident from the BiVO4 side, the BiVO4 layer mainly absorbs visible light with a wavelength of up to 520 nm, the WO3 layer transfers electrons efficiently, and the SnO2 layer reduces the charge recombination loss at the interface.
Figure 5 shows the current-voltage characteristics of the developed oxide photoelectrode. When water was decomposed in a high-concentration carbonate electrolyte by using the three-layered photoelectrode, the solar energy conversion efficiency was 0.85%. Stacking of two of the photoelectrodes to confine light increased the solar conversion efficiency to 1.35 % in the high-concentration carbonate electrolyte. This efficiency is the world’s highest among those of oxide photoelectrodes without noble metal and is about twice the reported highest value. When the water decomposes, hydrogen bubbles form on the counter electrode surface and oxygen bubbles on the photoelectrode surface. Use of the developed electrode can reduce the voltage for water electrolysis by more than 40 % and would lead to low-cost hydrogen production by water electrolysis.
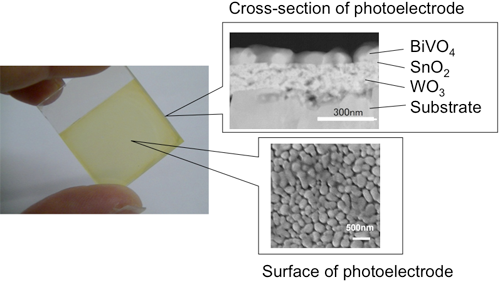 |
Figure 4 : Photo (left) and electron microscope images (left) of the multilayered photoelectrode |
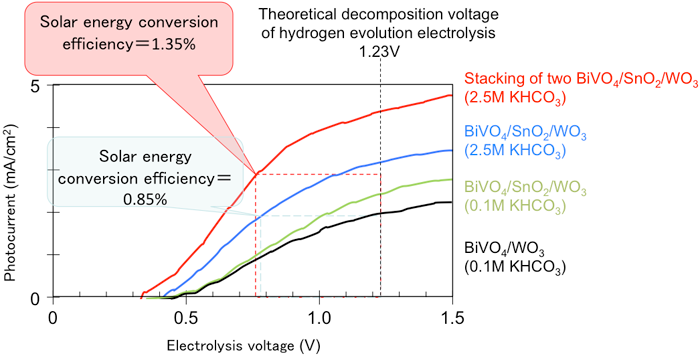 |
Figure 5 Current-voltage characteristics of the photoelectrode
Water can be decomposed at a low electrolysis voltage using solar energy. |
To improve the solar energy conversion efficiency of the photoelectrode, it will be necessary to further reduce the voltage of the auxiliary power supply while increasing the photocurrent. It is important to develop a semiconductor that has three features: the ability to fully use visible light with longer wavelengths; a negatively high conduction band level; and a high charge-separation efficiency. To achieve this goal, the researchers have developed a robotic system that can automatically search for promising semiconductors and suitable multilayered film structures from among numerous composite materials and their combinations in a short time. They have been using the system for high-speed screening. They will search for materials and improve the photoelectrode preparation method to increase the solar energy conversion efficiency. In the high-concentration carbonate electrolyte, carbonate ions are thought to promote the water electrolysis reaction just as a catalyst does by repeating oxidation-reduction cycles. They aim at the elucidation of this mechanism in detail in order to increase the efficiency of the water electrolysis system.