- Macroscopic properties of superconducting Josephson junctions change drastically with tiny differences in thickness of ferromagnetic insulators -
Shiro Kawabata (Senior Research Scientist) of Nanomaterials Theory Group, the Nanotechnology Research Institute (Director: Nobutsugu Minami) of the National Institute of Advanced Industrial Science and Technology (AIST; President: Tamotsu Nomakuchi) and his colleagues have discovered, from simulations of Josephson junction devices with a structure in which a ferromagnetic insulator is sandwiched between high-temperature superconductors (Fig. 1), a phenomenon whereby two macroscopic quantum states of a junction device (0 junction and π junction) are alternately realized when the thickness of the ferromagnetic layer is changed in increments of one atomic layer. The discovery that macroscopic properties over the whole device are changed drastically by differences in atomic-scale thickness is of great interest for basic science. On the basis of this discovery, we predict that macroscopic properties of superconducting devices can be artificially controlled by magnetic material thicknesses. We expect the application of such devices to lead to the future realization of quantum bits and quantum computers that are both robust to external noise.
Quantum computers have attracted great interest in recent years because of their potential for realizing massive parallel computation that greatly surpasses the capabilities of current computers. A major key to the realization of quantum computing is finding a way to eliminate the effects of external noise, which destroys quantum coherence. With the application of the phenomenon we have discovered, there is potential for realizing quantum bits that are completely unaffected by external noise and are capable of high-temperature operations. With such quantum bits, a possible road to practical quantum computers will be opened. In addition, we expect new applications to high-sensitivity magnetic sensors, quantum voltage standards, and suchlike.
This research was conducted as a part of the research project "Framework Development for Multiscale and Multiphysics Simulations toward Novel Applications of Superconductivity" (Research representative: Masahiko Machida) under the research area "High Performance Computing for Multi-scale and Multi-physics Phenomena" (Research leader: Genki Yagawa) of the Core Research for Evolutional Science and Technology (CREST) team research of Japan Science and Technology Agency.
The results of the research will be published in the electronic edition of Physical Review Letters, a scientific journal of the American Physical Society.
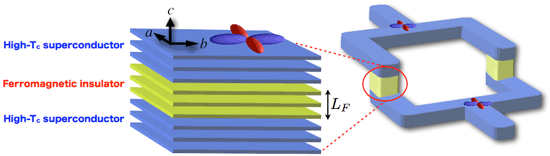 |
Fig. 1 A schematic drawing of a superconducting Josephson junction in which a ferromagnetic insulator is sandwiched, and a quantum bit. |
Quantum computers based on the principles of quantum mechanics will have both high-speed computing performance far outstripping conventional computers and very low power consumption. Accordingly, research into their realization is being advanced around the world. At the same time, various approaches for realizing a quantum bit that is a basic element of quantum computers are being developed.
Quantum computers need to maintain quantum coherence during computations. Therefore, the elimination of the influence of the external noise that interferes with quantum coherence is an important issue. Software-based error correction technologies such as quantum error correction have been developed, but these conventional technologies have problems in that it is necessary to add redundant quantum bits for error correction—five times the number of quantum bits used for computing or more—and this complicates fabrication and computing processes. The realization of physically robust quantum bits that are resistant to the effects of external noise is highly desired.
At AIST, in collaboration with experimental and theoretical research groups, we have been making progress with research and development of the application to quantum computing of both high-temperature superconducting Josephson junction devices and Josephson junction devices in which a magnetic material is sandwiched. We have also been developing simulation techniques for analyzing the transport properties of Josephson junction devices.
Now, using the simulation techniques developed in AIST, we have analyzed the transport properties of Josephson junction devices in which ferromagnetic insulators are sandwiched and achieved this success, in collaboration with: Yasuhiro Asano (Lecturer), Department of Applied Physics, Graduate School of Engineering, Hokkaido University; Satoshi Kashiwaya (Leader), Low-Temperature Physics Group, Nanoelectronics Research Institute, AIST; Yukio Tanaka (Associate Professor), Department of Applied Physics, Graduate School of Engineering, Nagoya University; and Dr. Alexander A. Golubov, the Low Temperature Division, University of Twente, The Netherlands.
It has been known since the late 1970s that π junction is realized in Josephson junction devices that sandwich ferromagnetic metals, such as iron. We analyzed the properties of Josephson junction devices that sandwich ferromagnetic insulators. For this analysis, we employed a simulation technique based on a discrete lattice model (a tight-binding model) that we had developed. In this technique, discrete Schrödinger equations describing electric states in a lattice model, as shown in Fig. 2, are solved to simulate the Josephson currents flowing through a device. The advantages of this technique include very broad applicability and the ability to deal with arbitrary device shapes and various magnetic and superconductive materials. Consequently, simulations based on realistic models are possible.
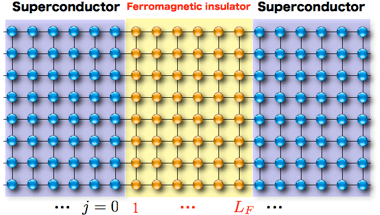 |
Fig. 2 A discrete lattice model used in our computer simulations of Josephson currents |
Figure 3 shows results of simulating Josephson critical currents with the thickness of a ferromagnetic insulator being varied at the level of single atomic layers. (The vertical axis in Fig. 3 shows absolute current values relative to the current value when the ferromagnetic insulator is one atomic layer thick. The red circles show negative values and the blue circles show positive values.)
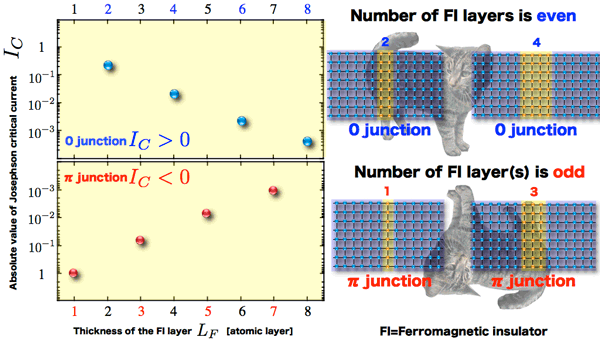 |
Fig. 3 Numerical results showing a π junction (negative Josephson critical currents) and a 0 junction (positive Josephson critical currents) being alternately formed when the thickness of the ferromagnetic layer is changed by one atomic layer (the vertical axis represents Josephson critical current values, which are absolute values relative to the current value when the magnetic layer is one atomic layer thick). These results show that slight differences in thickness—single atomic layers—produced dramatic changes in macroscopic states (e.g., as represented by the upright cat and the inverted cat). |
When the number of the ferromagnetic insulator layers is even, i.e. two, four, six or eight, the Josephson critical currents were found to have positive values, so the 0 junction is formed. More peculiarly, when the number of the layer(s) is odd, i.e. one, three, five or seven, the Josephson critical currents had negative values and the formation of π junction was predicted. This is the first theoretical prediction of the π junction formation in Josephson junction devices with ferromagnetic insulators. More interestingly, we showed the appearance of atomic scale 0-π transition by increasing the number of the ferromagnetic insulator layers. Our finding suggests that macroscopic quantum states over a superconducting device can be controlled by the tiny alteration (a local alteration) of adding or removing a single atomic layer. This is a quite interesting finding from the perspective of the basic science.
Regarding quantum computer applications, ferromagnetic insulator based π junction will be a basic component of an ideal quantum bit. In a superconducting ring including a π junction as shown in Fig. 4, a quantum superposition between 0 and 1 states, i.e. a quantum bit, is spontaneously generated, even without the application of an external magnetic field. With an ordinary superconducting magnetic flux quantum bit based on a similar superconducting ring with a 0 junction, an external magnetic field application is required to realize a quantum bit. However, the presence of noise in the applied magnetic field is unavoidable, and this noise destroys the quantum coherence. On the other hand, in a superconducting ring with a π junction, we do not need to apply the external field, and thus can realize a quantum bit that is unaffected by external noise. It should be noted that a similar quantum bit could be realized with a π junction using a ferromagnetic metal instead of a ferromagnetic insulator. However, this has a serious drawback, i.e., the excitation of thermal electrons in the metal layer destroys the quantum coherence. Therefore, it is difficult to realize a coherent quantum bit using a ferromagnetic metal. In contrast, there is no problem of the excitation of thermal electrons in the case of a ferromagnetic insulator, so an ideal quantum bit based on ferromagnetic insulators is possible.
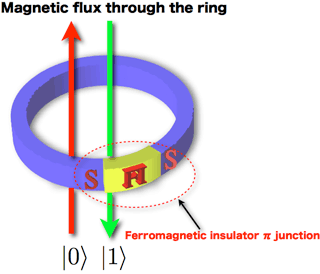 |
Fig. 4 A π junction quantum bit using a superconducting ring. S indicates a superconductor and FI indicates a ferromagnetic insulator. A quantum bit (a state in which 0 and 1 are superposed) is spontaneously formed even without the application of a magnetic field. Therefore, there is no effect from the external noise associated with a magnetic field. The 0 and 1 states here correspond to quantum states in which magnetic flux passes through the ring upward and downward, respectively.
|
We also simulated a Josephson junction device with a three-dimensional structure made of a high-temperature oxide superconductor and a ferromagnetic oxide because such atomic-scale oxide junctions can be realized experimentally by use of current fabrication technologies (Fig. 1). We confirmed that the 0 and π junctions could be formed alternately when thickness of a ferromagnetic oxide layer is increasing by a single atomic layer. Therefore, we can expect the realization of a quantum bit that operates even at temperatures one or two orders higher than conventional superconductor quantum bits (1 K and above). This could possibly lead to the realization of practical quantum computers.
In the future, we will incorporate the effects of imperfections in the structures of Josephson junction devices and perform more realistic simulations to optimize device properties. We will also collaborate with experimental groups in universities and research institutions both in Japan and abroad with the aim of realizing quantum bits. We will also try to find new applications, e.g., high-sensitivity magnetic sensors, quantum voltage standards, and suchlike based on our findings.