- Elucidation of a chemical reaction of fullerene molecules by high-resolution electron microscopy -
Masanori Koshino (Research Scientist), the Nano-carbon Characterization Team (Leader: Kazutomo Suenaga) and Toshiya Okazaki (Senior Research Scientist) of the Nanotube Research Center (Director: Sumio Iijima), Hiromichi Kataura (Leader), the Self-Assembled Nano-Electronics Group of the Nanotechnology Research Institute, the National Institute of Advanced Industrial Science and Technology (AIST) (President: Tamotsu Nomakuchi), Yoshiko Niimi (Research Technical Assistant), the Japan Science and Technology Agency (JST), and Eiichi Nakamura (Professor), the Department of Chemistry, Graduate School of Science, the University of Tokyo, have jointly succeeded in the atomic level analysis of the reactivity and selectivity of the dimerization reaction of fullerene molecules.
In this study, the group confined the fullerene molecules in single-walled carbon nanotubes and optimized the chemical reactivity of the molecules by changing parameters such as concentration, temperature, effects of metal atoms and energy imparted, thereby visualizing the chemical reaction of the molecules under a high-resolution electron microscope with an aberration correction system. The visualization revealed that the orientation of each molecule directly affected the reaction. It is expected that this technique has a wide variety of applications including elucidation of various reaction mechanisms and molecular design, e.g., in the development of new drugs.
The results were obtained from the ERATO research project titled "Nakamura Active Carbon Cluster Project" (Research General Manager: Eiichi Nakamura) and the research theme "Development of low-acceleration and high-sensitivity electron microscope enabling observation of soft matters at molecular and atomic levels" (Research representative: Kazutomo Suenaga) in the CREST research field titled "New measurement and analysis base technique contributing to elucidation and application of material phenomena" (Research General Manager: Michiyoshi Tanaka, Emeritus Professor, Tohoku University); the projects were implemented under the Basic Research Programs of JST. The details of the research will be published in an online edition of a British scientific journal, "Nature Chemistry," at 3 am, January 11, 2010 (Japan standard time).
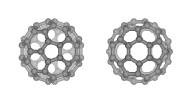 |
Model of C60 fullerene
At which vertex or on which side of the pentagon and hexagon will two molecules be combined? |
Chemical reactions range from familiar reactions that are indispensable for life activities such as respiration and digestion to those are essential for modern industry, such as chemical syntheses and energy conversion. The reactive part of a molecule can be predicted theoretically to some extent, but this prediction is very difficult in some actual chemical reactions. One example is the fusion of fullerene molecules in which different chemical reactions occur simultaneously and various products are obtained. Since conventional chemical analyses were based on the statistics to analyze the average behavior of large numbers of molecules, they were not effective for examining the reactions that produce mixed various products. On the contrary, it is believed that the new technique of nanotechnology enables us to analyze the behaviors of individual molecules and to clarify their reactions. Atomic information during the course of chemical reactions is expected to elucidate several unknown phenomena. This is the reason why such new analyses of nanotechnology have been demanded and developed.
Organic molecules are composed of light elements. It was very difficult to observe the behavior of such light molecules at the atomic level. In recent years, however, it has become possible to observe the behavior of one small organic molecule by confining it in a carbon nanotube with nanometer-sized internal space. It has been proved that this confinement technique can be applied to the analyses of molecular behaviors. One of the next goals is to visualize each molecule involved in a chemical reaction at the atomic level. The visualization is expected to provide knowledge that has not been acquired by using other analysis methods, to contribute to the understanding of chemical reactions at the atomic level, and to elucidate reaction mechanisms.
The group has succeeded in observing the reaction processes of fullerene molecules confined in carbon nanotubes at the atomic level by using an electron microscope with a low acceleration voltage and an aberration correction system, in combination with fast-Fourier-transform image processing. The development of the electron microscopy enabled high-resolution observations under various environments such as ultra low temperatures (-269 °C) and low acceleration voltages (80 kV). The results indicate that molecular behaviors and chemical reactions could be controlled.
It is known that the irradiation of two fullerene molecules by an electron beam causes a dimerization to occur. In this study, fullerene molecules (diameter: 0.7–0.8 nm) were confined in carbon nanotubes (inner diameter: 1.2–1.5 nm) to observe the dimerization between the molecules at the atomic level by using an electron microscope with an aberration correction system.
As shown in Fig. 1, a transmission image of C60 fullerene molecules confined in the carbon nanotube is obtained using the electron microscope, looking like a transparency. As the resolution of the microscope is improved, a striped pattern of the nanotube overlaps with the intrinsic patterns of fullerene molecules, which hinders structural analysis of the fullerene molecules. By mathematical processing (e.g., calculating the fast Fourier transform) of this image, the striped pattern of the tube could be removed, and the patterns of the fullerene molecules could be revealed. This enabled us to observe the molecules at the atomic level. The two lines above and below the fullerene molecules represent the walls of the carbon nanotube.
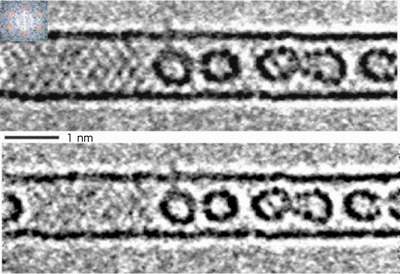 |
Fig. 1 Electron microscope image of C60 fullerene molecules confined in a carbon nanotube (upper) and image without the striped pattern of the nanotube (lower) |
Figure 2a shows electron microscope images of the change in C60 fullerene molecules; the molecules were irradiated with an electron beam and fused together by a dimerization. The dimerization proceeded from left to right when the same molecules were irradiated with the beam. Patterns within the molecules are revealed in the images shown in Fig. 2b, where the contrasts (light and dark) in the images in Fig. 2a are emphasized. The model images in Fig. 2c show how the molecules face each other during the reaction.
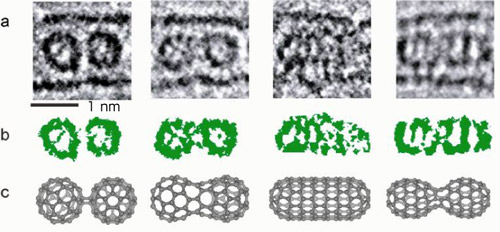 |
Fig. 2 (a) Electron microscope images of the C60 fullerene molecules
The molecules were irradiated with an electron beam and fused together by a dimerization. The electron dose increased from left to right, and the chemical reaction proceeded.
(b) Image emphasizing contrasts (light and dark) of the molecules
(c) Model structures of the molecules |
There were no analysis methods that could be used to obtain information during the course of the chemical reaction, for instance, which faces of the molecules are combined first, whether they fuse together on a pentagon or hexagon of the C60 fullerene molecules, or whether carbon atoms at the vertex fuse together. An actual image obtained using the microscope (leftmost image in Fig. 2a) was compared with a computer-simulated electron microscope image. The result indicated that some patterns of the combinations between the molecules can be predicted. Although many structures of the combinations could be assumed, a model structure of [2 + 2] cyclic condensation shown in Fig. 2c was found to agree well with the observed images. A side between the pentagon and hexagon of the molecules to the left and one between the hexagons of the molecules to the right formed cyclic bonds.
When the reaction proceeded further with the gradual irradiation by the electron beam, a peanut-shaped structure generated via the fusion of C60 fullerene molecules was observed (second from the left in Fig. 2a). For this structure, five different model structures were assumed. Judging from the contrast in the image of the molecules, one of these five structures was selected (second from the left in Fig. 2c).
By the further irradiation, the peanut-shaped molecule changed to a tube-shaped molecule (second from right in Fig. 2a). It is known that the tube exhibits metallic or semiconducting properties according to its chirality (mesh pattern). The contrast in the image of the observed tubular molecule revealed the tube to be of zigzag type exhibiting metallic properties. Finally, the tubular molecule was changed to a peanut-shaped molecule (rightmost in Fig. 2a) which is slightly larger than the first peanut-shaped molecule, but the contrast of the molecule agreed well with that in a simulation image obtained using the model structure.
As described above, with the progress of the dimerization of the C60 fullerene molecules, the change in the electron microscope images of the two fullerene molecules could reveal how the molecules interact with each other after they first make contact, as well as how they fuse together with the reconstitution of chemical bonds.
In addition to these behaviors, the effects of various external factors on the chemical reactions were examined in detail. The factors include the temperatures, the number of molecules confined in the carbon nanotubes (concentration), presence or absence of metal atoms during the reaction, and the applied energy used in the chemical reactions. The result demonstrates that chemical reactions at the atomic level could be visualized under various experimental conditions.
Atomic-level observation of chemical reactions and the control of factors related to the experimental environment, such as temperatures, concentrations and directions of molecules, presence of metal atoms in the molecules, and the amount of energy supplied to the reactions, enables us to control and analyze the reactions of individual molecules. The future application of this technique to organic molecules and biomolecules is expected to elucidate the mechanism of reactions of individual molecules critical to life processes, enable the dynamic analysis of molecular interactions, and aid development in a wide variety of research fields, including molecular design based on structural chemistry, e.g., in the development of new drugs.