- a highly promising material for applications such as photodeterioration inhibition -
Hiromichi Kataura (Leader) and Kazuhiro Yanagi (Research Scientist) of the Self-assembled Nano-electronics Group, the Nanotechnology Research Institute (Director: Hiroshi Yokoyama) of the National Institute of Advanced Industrial Science and Technology (President: Hiroyuki Yoshikawa) (hereinafter referred to as AIST) measured the ability of each nanocarbon material to remove singlet oxygen, a kind of active oxygen species. In cooperation with Toshiya Okazaki (Senior Research Scientist) of AIST’s Research Center for Advanced Carbon Materials (Director: Sumio Iijima), they discovered that higher fullerene and endohedral metallofullerene can deactivate singlet oxygen with great efficiency.
Because singlet oxygen is highly active, it is closely related to the deterioration of various substances exposed to light. It greatly affects a number of aspects of our lives, for example by damaging DNA in the living body and contributing to skin deterioration. This is why substances with the ability to remove singlet oxygen are vital from the viewpoints of both photodeterioration control and preventive medicine.
Although nanocarbon materials are expected to have various applications, they have never been studied as materials for the removal of singlet oxygen. In this research, we performed detailed measurements and verified the ability of nanocarbon materials to remove singlet oxygen. We found that higher fullerene C82 and endohedral metallofullerene have the same excellent removal ability as carotenoid pigment (beta carotene in this research), the substance with the highest removal capacity in nature. Fullerene is excellent because it can be mass-produced and processed at high temperature, and it can be thinned with the help of vacuum deposition.
AIST has been asking companies with related technologies to participate in this research in order to establish applications for this material as a photooxidation inhibitor and in preventive medicine. Details of the results will be published in “Nano Tech 2007”, scheduled for 21–23 February at Tokyo Big Sight.
Singlet oxygen—a form of active oxygen—is an excited-state oxygen generated by exposure to light. Excited-state oxygen is a factor involved in the photodeterioration of materials because it is highly responsive and able to oxygenize various kinds of materials easily. In addition, it greatly affects various aspects of our life by, for example, injuring genes (DNA) and causing deterioration of skin in the living body. Fullerene C60 (a basket-shaped molecule made up of 60 carbons)—a representative nanocarbon material—is known to generate singlet oxygen highly efficiently upon ultraviolet exposure.
This is because C60 energy (energy in the triplet state) generated through excitation by light is transferred to oxygen and changes to active singlet oxygen (Figure 1a). However, the level of excited-state energy decreases with the number of carbon atoms in the cage of higher fullerenes. Accordingly, in large high-order fullerenes, the excited-state energy declines to a level below that needed to transform oxygen to singlet oxygen, making it possible to deprive the singlet oxygen of energy and thus cause it to revert to the ground state of oxygen (Figure 1b). By enlarging the size of the package systematically, we therefore decided to examine the ability of a series of fullerenes to remove singlet oxygen.
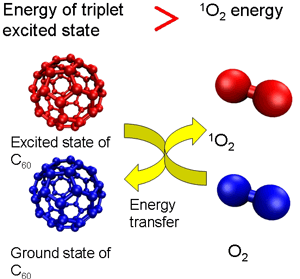
Figure 1 (a)
|
|
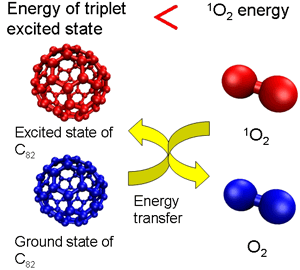
Figure 1 (b)
|
Fullerene C60 is famous, as is the above-mentioned high-order fullerene. In addition, endohedral metallofullerene, in which metal atoms are sealed inside a fullerene basket, is also well known. In this research, we systematically measured the singlet oxygen removal capability of both fullerenes with different basket sizes and metal-packaged fullerenes such as lanthanum (La) and cerium (Ce)-packaged fullerene. We also measured the capability of carbon nanohorn, which has a horn-shaped structure on the tip.
To measure the constant of the singlet oxygen deactivation rate constants of the sample (kq + kr), we generated singlet oxygen by using a molecule that generates singlet oxygen by heat. We then measured the intensity of emission from the singlet oxygen in the organic solvent, and we estimated the decrease in the emission intensity through the addition of the sample (Figure 2). The greater the value, the greater the ability to cause singlet oxygen to revert to the ground state of oxygen. We compared these values with the data on carotenoid pigment (in this case beta-carotene), which is known to have the biggest removal capability in nature.
Fullerene C60 is known to generate singlet oxygen with high efficiency upon ultraviolet light exposure, and its energy in the excited and triplet state is larger than the energy of singlet oxygen. Reflecting this fact, the singlet oxygen removal capability of the molecules in C60, C70, C76 was very small—smaller than the measurement sensitivity.
In contrast, however, fullerene (C82) with a bigger basket size removed singlet oxygen with high efficiency. Endohedral metallofullerene had an even higher removal capacity that was the same as, or more than, that of beta-carotene. This is supposedly because, in fullerene with a high removal capacity, the energy level in the excited state (excited triplet and doublet) is lower than that of singlet oxygen; it deprives the singlet oxygen of energy, making it revert to the graound state of oxygen.
It has been clarified for the first time that carbon nanohorn also has singlet oxygen removal ability. This graphite structure with a large curvature like that of a horn tip is estimated to respond readily to active oxygen. Unlike the removal mechanism of carbon nanohorn, singlet oxygen is supposed to react with the graphite structure.
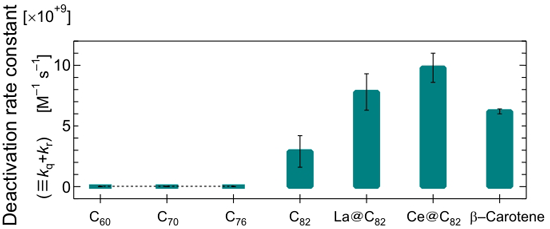 |
Figure 2
|
We are continuing to work on an inhibitor of the photooxidation that is one of the causes of deterioration of materials exposed to ultraviolet light. These days the need to verify the safety of nanocarbon materials has attracted public attention. We will pursue the possibility of using nanocarbons as long-life photooxidation inhibitors from a structural viewpoint, while at the same time confirming their safety. In the future, we will develop applications not only in the materials field but also in the biological and medical fields.