- A 2-quantum bit computing device enabling ultra-high speed (pico-second step) operation -
The National Institute of Advanced Industrial Science and Technology (AIST, President: Hiroyuki Yoshikawa) has successfully developed the world's first basic device structure extendable to multi-quantum-bits for an optically controlled quantum logic gate. This achievement is part of the "Core Research For Evolutional Science and Technology (CREST)" project of the Japan Science and Technology Agency (JST).
Quantum computers are expected to be able to process huge amounts of information so that they can rapidly solve problems that take even supercomputers a long time. Because of this, organizations in many countries have been working on their development. Basic devices for quantum computers must have a structure enabling small size and multi-bit integration.
Recently, many studies on quantum bits (qubits) and quantum logic gates using solid-state materials such as superconductors and semiconductors have been reported. For devices utilizing excitons (electron-hole pairs) in semiconductors, optically-controlled ultra-high speed processing (a computing step of pico-seconds) is possible. However, there have been no reports on basic device structures which enable both "multi-bit" and strong "interaction between the two qubits," which are essential for quantum logic gates. Thus, recent work has focused on developing such quantum logic gates.
In this study, we prepared coupled quantum dots, which are formed by closely arranging 2 or more quantum dots. By utilizing excitons confined in the respective dots as the qubit, we were able to create multi-bit structures. Furthermore, we have designed a unique structure which can induce strong interactions between the two qubits by adequately adjusting the distance between the dots.
Also in this study, using the MBE technique, we have fabricated a very small dot structure coupled in the direction of stacking, and developed a technique which can optically control and detect two separate excitons in the coupled dots. Furthermore, using these techniques, we have successfully developed the world’s first optically controlled 2-qubit logic gate structure capable of having multi-qubits and strong interactions between the two qubits (see Figures 1, 2 and 5).
The results of this study appear in the latest issue (December 19) of Applied Physics Letters.
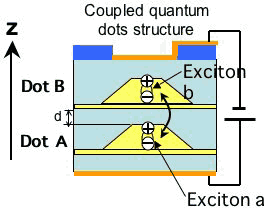
Figure 1 Schematic diagram of a 2-qubit logic gate using two excitons in coupled quantum dots. |
|
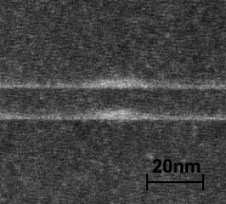
Figure 2 A cross sectional TEM image of coupled quantum dots, where InAs quantum dots of 20 nm in diameter and 3 nm in height are coupled with each other through a GaAs barrier.
|
Quantum computers have been expected to be able to process a huge amount of information so that they can quickly solve problems that take even supercomputers a long time to answer. While conventional computers use "bits" having a value of 0 or 1 as the minimum unit of data, quantum computers use a 2-level system which is able to additionally take a superposition of 0 and 1 states as "qubits."
For conventional computers, logic operations are constructed using combinations of NOT and AND gates. Similarly, for quantum computers, it has been theoretically proved that quantum logic operations can be constructed using a 1-qubit logic gate (rotation gate) and a 2-qubit logic gate (controlled NOT or controlled rotation gate). Thus, the fabrication of these two types of quantum logic gate is the first step toward the realization of quantum computers.
In the development of quantum logic gates, studies on devices using atomic and molecular systems (ion trapping, NMR, etc.) were first carried out, and an experimental 7-qubit quantum algorithm calculation has been reported. However, for devices with atomic and molecular systems, the qubit number is limited to be at most 10, indicating that the execution of practical algorithms is difficult. Thus, devices using solid-state materials such as superconductors and semiconductors, which enable small size and multi-bit integration, have been actively studied.
In the case of solid-state quantum logic gates, many examples of 1-qubit devices, but only two examples of 2-qubit devices and their device operation, have been reported. One is an electrically controlled device utilizing charges in a superconductor, and the other is an optically controlled device utilizing excitons in a semiconductor nanostructure. This is because such devices are very difficult to fabricate and to put into device operation.
Although the latter optically controlled device has an outstanding feature capable of ultra-high speed operation (1 operation step is subpico-seconds to pico-seconds), it has been difficult to combine both a "structure enabling multi-bit integration" and "strong interactions between the two qubits necessary for operation," which are essential for quantum logic gates. So far, one example has been reported, but it is only a demonstration of the preliminary device operation in a structure which cannot fabricate multi-bit devices in principle.
In this study, we have prepared coupled quantum dots which are formed by closely arranging two or more quantum dots. By utilizing excitons confined in the respective quantum dots as a qubit, we have made multi-qubit integration possible. In addition, we have proposed a unique structure which can induce strong interactions between the two qubits by adequately adjusting the distance between the dots to overlap the electron wavefunction of the exciton in one quantum dot with that in the neighboring quantum dot.
Also in this study, we have developed a technique to fabricate a very small coupled quantum dots structure, and a technique which can optically control and detect the state of two separate excitons in the coupled quantum dots. Furthermore, using these techniques, we have successfully formed the world’s first 2-quamtum bit state, "11", which requires strong interactions (an energy shift of approximately 1.3meV) between the two qubits, i.e., two excitons. This strongly indicates that the device structure we have developed can be utilized as an optically controlled 2-qubit logic gate.
AIST has developed ultrahigh-speed (sub-pico second) optical devices for next-generation optical communications and quantum information devices for future quantum communications and quantum information processing technology. In the present study, we used the most advanced fabrication techniques for high-quality semiconductor quantum nanostructures and devices to construct the world's first 2-qubit logic gate structure which utilizes excitions in coupled quantum dots.
The results of this study have been achieved with the support of JST as part of the "Coherent Quantum-Control and Information-Processing Technology" study theme in the research area "Creation of Nanodevices and Systems Based on New Physical Phenomena and Functional Principles" undertaken under the "Core Research for Evolutional Science and Technology (CREST)" project.
The 2-qubit logic gate we developed in this study features a coupled quantum dot structure, which is formed by placing two semiconductor quantum dots (A and B) close together in the stacking direction, and one exciton for each dot, denoted as excitons a and b. These excitons, which have different excitation energy (wavelengths), are used as qubits.
Figures 1 and 2 show the structure of a quantum logic gate using coupled quantum dots. Figure 3 shows an excitation level diagram for a 2-qubit device. The "0" and "1" states of the qubit correspond to the states where the an exciton is not excited (a crystal ground state) and is excited (one exciton state), respectively. Hereafter, the four states of excitons a and b are defined as "a, b" = "00", "01", "10", "11".
In our device, the 1-qubit operation (1-qubit rotation gate operation) is achieved by changing the state of exciton (a) from 0 to 1, or from 1 to 0, or to a superposition of 0 and 1 states using an optical pulse with a transition wavelength (transition energy: Xa) of exciton (a).
Also, for the 2-qubit operation (controlled rotation gate operation), exciton (a) is assigned to a control bit, and exciton (b) is assigned to a target bit. When exciton (a) is in the state "1" and exciton (b) is created as "1", an interaction can be induced between the excitons (or interaction between the qubits). Thus, utilizing this induced energy change of ΔE, the controlled rotating gate operation can be achieved, but only when exciton (a) is in the state "1".
Specifically, when exciton (a) is in the state "0", photon energy to excite exciton (b) is denoted by Xb ("00" ⇒ "01" in Figure 3); when exciton (a) is in the state "1", the photon energy to excite exciton (b) is decreased by the interaction energy of ΔE between excitons a and b to become Xb' = Xb - ΔE ("10" ⇒ "11" in Figure 3). Thus, only when exciton (a) is in the state "1" can it conditionally achieve the controlled rotating gate operation, where the state of exciton (b) is rotated from 0 to 1 (or from 1 to 0 or superposition states of 0 and 1) using an optical pulse with an energy of Xb'.
Since the optical pulse has an energy broadening (∝1/ pulse width), if the energy difference ΔE between Xb and Xb' is smaller than the energy broadening of the pulse, conditional rotating gate operation is impossible. Thus, the interaction energy (ΔE) must be sufficiently larger than the energy broadening of the control pulse. For our optically controlled device, the energy broadening of the control pulse has a large value of 0.4 meV (corresponding to a 5 psec pulse), so the interaction energy of ΔE must be sufficiently larger than 0.4 meV.
The figure of merits of our proposed structure of the 2-qubit quantum logic gate is as follows. By adequately adjusting the distance between the quantum dots (d in Figure 1), if we choose the strongly coupled structure with the barrier thickness, d, of about 5nm, a hole of the exciton can be localized in each quantum dot, while a part of the electron wavefunction of the exciton in one quantum dot can be overlapped with that in the adjacent quantum dots to induce interactions between the qubits. Thus we can realize both a relatively strong interaction between the two qubits, and the selective excitation of the exciton, enabling simultaneous multi-bit integration.
In the experiment, using the MBE technique, we have fabricated an InAs/GaAs quantum dot structure coupled in the direction of stacking. Furthermore, in order to irradiate the optical beam to only one pair of quantum dots, we created a metal mask (or micro pillar structure) having a sub-micron aperture of 0.2 µm in diameter and an electrode structure to apply an electric field (Figures 1 and 2).
An experimental result of the measurement of the 1-qubit state of the devices, i.e., low temperature photoluminescence (PL) characteristics under the condition of low optical excitation, is shown in Figure 4. The photoluminescence spectrum consists of peaks at photon energies of Xa (for exciton (a)) and Xb (for exciton (b)) which are used as control bit and target bit, respectively, and peaks at higher photon energies of X2a and X2b are due to the hole levels of excitons a and b, respectively.
To determine whether or not the fabricated devices satisfied the requirement for 2-qubit controlled rotation gates, we investigated whether the 2-bit states "00", "01", "10", and "11" could be created and controlled separately or not.
Figure 5 shows the photoluminescence characteristics of coupled quantum dots when excitons (a) and (b) are excited independently with two wavelength lasers. The lower curve shows that a state of exciton (a), "10" (with photon energy of Xa), can be independently created.
The central curve shows that a state of exciton (b), "01" (with photon energy of Xb), can also be independently created. The upper curve in Figure 5 shows that when the excitons (a) and (b) were excited simultaneously by two wavelength lasers. A new PL peak, resulting from the interaction between excitons (a) and (b), appeared at a photon energy of Xb', which is shifted from Xb by ΔE. This observation is evidence that the creation of the 2-qubit state "11" (with photon energy of Xb') is possible.
To further confirm this finding, we examined the intensity of the photoluminescence emitted from the 2-qubit state as a function of the pumping optical power of both the Xa exciton and the Xb exciton (x- and y-axes). As a result, we again confirmed the formation of the 2-qubit state "11" as shown in Figure 6.
Furthermore, the interaction energy (i.e., the energy difference between Xb and Xb') was found to be 1.3 meV, which is sufficiently larger than the energy broadening of the control optical pulse of about 0.4 meV required for ultra-high speed optical control. This shows that our device structure can be utilized as a 2-qubit quantum logic gate, and as such is the world's first demonstration of a 2-qubit solid state device structure that can enable both an ultrafast optical control and multi-bit integration.
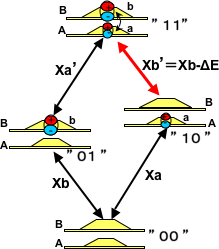
Figure 3 An excitation level diagram of the 2-qubit logic gates using excitons. The relations "input ⇒ output" of controlled rotating gate operation are shown as "00" ⇒"00", "01" ⇒"01", "10" ⇒ -"11", and "11" ⇒"10".
|
|
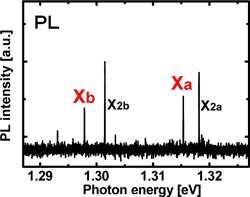
Figure 4 Photoluminescence of Xa from exciton (a) and of Xb from exciton (b), which are utilized as control qubit and target qubit, respectively. Photoluminescence at X2a and X2b is due to the excited states of the respective holes in the exciton.
|
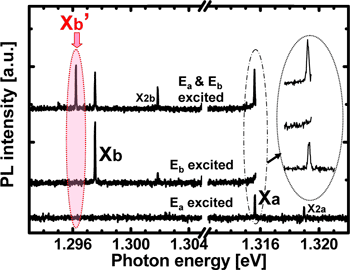 |
Figure 5 Photoluminescence of Xa (bottom) and Xb (middle) when exciton (a) and exciton (b) are separately excited. Photoluminescence from a new 2-qubit state "11" (with photon energy of Xb') was observed only when both excitons a and b were excited one by one (top), indicating the creation of a 2-qubit state due to a interaction between the two qubits.
|
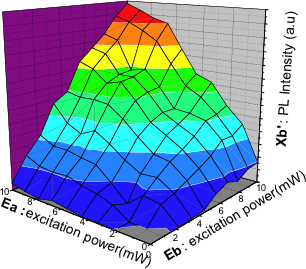 |
Figure 6 An experimental result showing the generation of a 2-qubit state of "11" by creating two exciton qubits, (a) and (b), one by one. The intensity of photoluminescence at a photon energy, Xb', increases in proportion to the each optical power to create excitons (a) and (b), respectively. This is the first demonstration of the formation of a 2-qubit state "11" by interaction between the two qubits (excitons) confined in the different quantum dots.
|