Dr. Zhou Haoshen, a senior researcher scientist, and his colleagues at the Energy Technology Research Institute (ETRI) of the National Institute of Advanced Industrial Science and Technology (AIST), an independent administrative institution, have demonstrated that using crystalline-glass mesoporous nanocomposite (CGMN) with nano-channel structures of 3D regular orientation for the negative electrode of lithium rechargeable battery makes it possible to keep the energy density at a level of the conventional lithium rechargeable battery, while increasing power density by two orders of magnitude or more.
The development of electrical vehicle (EV) powered by efficient and clean energy sources such as fuel cell and rechargeable battery is the target of heated competition throughout the world. While the lithium rechargeable battery is capable of sustained energy supply required for constant rate driving with adequate energy density, it is difficult to provide great power needed for quick start instantaneously. It is urgently demanded, therefore, to implement a storage device to provide adequate energy both in quick release (power density) and in sustained supply (energy density).
There are two approaches for realizing both high energy density and high power density simultaneously: (1) upgrading energy density through the combination of electrical double layer capacitor with pseudo-capacitor (super-capacitor technique) and (2) increasing power density of the lithium rechargeable battery itself. Both approaches have been tried since earlier, but no success has been achieved in drastic enhancement of the performance.
The research team at the ETRI-AIST has attempted to apply to the negative electrode of lithium rechargeable battery newly developed the crystalline-glass mesoporous nanocomposite, TiO2-P2O5, (AIST Press Release February 4, 2004), and demonstrated that the transport of lithium ions (Li+) and electrolyte solution is facilitated by oriented nano-channel structures and that the chemical adsorption/desorption of lithium ion at enormous surface area of nano-channels realizes super-capacitor function to ensure the energy density as high as that of the conventional lithium rechargeable battery and to upgrade the power density by two orders of magnitude or more. Furthermore, providing electronic conduction property to the framework by incorporating electronic conductive oxides into the framework of TiO2-P2O5 CGMN significantly improves the charge-discharge characteristics.
The future R&D efforts will be focused on the mass synthesis of this material and the cost reduction, aiming at the commercial application.
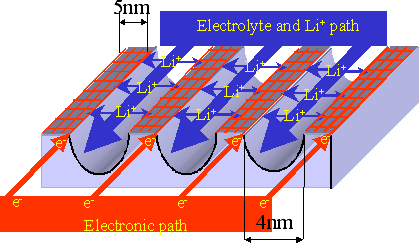 |
Figure An image of electrode material made from crystalline-glass mesoporous nanocomposite with 3D regularly aligned nano-channel structure equipped with both ion transport path and electron conduction path (schematic). |
The result of this work was published on Science Magazine Angewandte Chemie International Edition, 44, 2005, 797(web released on December 21, 2004)
The development of electrical vehicle (EV) powered by fuel cell or rechargeable battery as an efficient and clean energy source emitting less carbon dioxide, which causes global warming, has been actively pursued worldwide. It is rather difficult, however, to secure adequate accelerating or hill-climbing performance with fuel cell or rechargeable battery of limited quick release capability (power density). An energy storage device such as rechargeable battery with high power density and high energy density, if made available, will open the way in the application not only in EV but also in industrial and nursing care robots, notebook PC, mobile phone and other hi-tech areas, promising an enormous market in the future.
Electrical double layer capacitor (EDLC) with carbon fiber electrode of great surface area has been known as a storage device of high power density. The EDLC provides quick discharge-recharge sequence because of reversibly storing electrical charge through physical absorption-desorption of ions in electrolyte solution within the electrode, without relying on chemical reactions. This keeps the energy density of EDLC necessarily at a lower level. The EDLC is characterized by energy density 1 Wh/kg and power density 1 kW/kg. In view of application to EV, it is necessary to boost both energy and power density by an order of magnitude or more.
Recently, another approach for raising both energy density and power density has been proposed. In this approach, a pseudo-capacitor based on electrochemical reactions, storing ions through redox reactions, is created at the surface of electrode materials of rechargeable battery with an overwhelming advantage in respect to energy density, simultaneously realizing two merits: superb energy density of the rechargeable battery, and high power density of super-capacitor. The pseudo-capacitor makes it possible to discharge and recharge at a sufficiently fast rate owing to chemical adsorption and desorption of ions at the electrode surface, and yet, has great energy density. This is an ideal property for storage device of EV. However, the development of such a storage device is facing a bottleneck owing to unsuccessful development of porous electrode with nano-channel construction.
Development and Application of Mesoporous Nanocomposite Materials Based on Crystalline Metal
Oxide Materials
Based on an original concept, the ETRI-AIST has succeeded in synthesizing crystalline-glass mesoporous nanocomposite TiO2-P2O5 (Fig. 1), consisting of a crystalline phase and an amorphous phase and based on crystalline metal oxide equipped with 3D regular-aligned nano-channel structures, by adding a trace amount of an organic metal compound, trimethyl phosphoric acid, PO(OC2H5)3 to the synthetic process of metal oxide through the conventional template method. Moreover, the CGMN materials based on multiple crystalline metal oxide afforded with electron conductivity (TiO2-P2O5-CuO and TiO2-P2O5-SnO2) have been successfully synthesized by doping conductive cupric oxide (CuO) and stannic oxide (SnO2) into the three dimensional glass network in the framework of CGMN, AIST Press Release, February 4, 2004.
The ETRI-AIST has demonstrated that using the CGMN materials based on crystalline metal oxide for the negative electrode of lithium rechargeable battery facilitates the transport of lithium ion (Li+) and electrolyte solution into the interior of electrode through the oriented nano-channel construction of 5 nm size, and the adsorption of lithium ion (Li+) on enormous surface area of nano-channels realizes the super-capacitor features, allowing to keep the energy density as high as the level of the conventional lithium rechargeable battery and to upgrade the power density by two orders of magnitude or more. Additionally, it has been confirmed that providing electron conductivity to the framework constituting TiO2-P2O5 nano-channels will effectively upgrade the discharge-recharge characteristics.
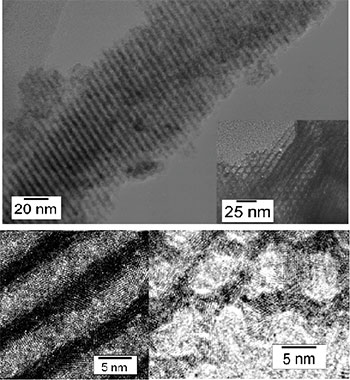 |
Fig. 1 Transmission electron microgram of micro-crystalline TiO2-P2O5 nano-porous powder with 3D construction
|
Battery Performance of Mesoporous Nanoconposite Material Based on Crystalline Metal Oxide
(TiO2-P2O5)
With the CGMN material based on crystalline metal oxide (TiO2-P2O5) used for the negative electrode of lithium rechargeable battery, the performance of the battery was evaluated. We only take TiO2 as active materials because both the specific capacities of pure AB and pure P2O5 are too small to be measured at a current density of 10A/g. Theoretically, the maximum capacity of anatase TiO2 is 165 mAh/g. It was demonstrated, however, that the new material could have much greater capacity than the theoretical maximum, for the constant current charge-discharge at 0.1 A/g current density, and could reach the capacity limit of reversible charge-discharge, 370 mAh/g (black line in Fig. 2). This data cannot be accounted for by the insertion/extraction process of lithium ion (Li+) into anatase TiO2, suggesting chemical adsorption and desorption of Li+ at the surface of nano-channels in the composite material (TiO2-P2O5). The mechanism of super-capacitor feature seems to be attributed to the chemical adsorption and desorption. The newly developed material is characterized by much higher energy density than that of the conventional anatase scheme, owing to simultaneous implementation of redox capacity and adsorption capacity.
In addition to drastic increase of the capacity (or energy density), the power density is also upgraded by two orders of magnitude or more. With the current density (or power density) for charge-discharge increased by two orders of magnitude from 0.1 A/g to 10 A/g, the charge-discharge capacity density (energy density) is kept as high as 270 mAh/g (red line in Fig. 2). The cycle performance, that is, durability for repeated charge-discharge operation cycles, is also improved significantly, keeping the capacity for charge-discharge with 10 A/g current density, 190 mAh/g after 200 cycles, and 160 mAh/g even after 800 cycles.
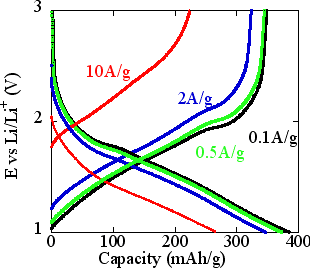
|
Fig. 2 The 2nd cycle charge-discharge profiles of TiO2-P2O5 CGMN in the potential range from 1.0 V to 4.0 V (vs. Li+/Li) with current densities 0.1 A/g, 0.5 A/g, 2 A/g and 10 A/g on the basis of active TiO2.
|
Battery Performance of Mesoporous Nanocomposite Materials Based on Multiple
Crystalline Metal Oxide (TiO2-P2O5-CuO and TiO2-P2O5-SnO2)
Furthermore, the battery performance of the CGMN materials based on multiple crystalline metal oxides (TiO2-P2O5-CuO and TiO2-P2O5-SnO2) doped with electron conductive functional substance CuO or SnO2 into the three dimensional glass P2O5 network in the framework of CGMN, was evaluated. It was confirmed that the high charge-discharge capacity density (or energy density) was maintained owing to the improved electron conductivity. For instance, with current density 20 A/g, the charge-discharge capacity density of TiO2-P2O5 was 60 mAh/g (green line in Fig. 3 bottom), and that of two composites with electron conduction path was: 190 mAh/g for TiO2-P2O5-CuO (blue line in Fig. 3 bottom), and 265 mAh/g for TiO2-P2O5-SnO2 (red line in Fig. 3 bottom). Even with still higher current density 50 A/g, the newly developed materials (TiO2-P2O5-SnO2) held as high capacity as 130 mAh/g (black line in Fig. 3 top).
When the CGMN material based on crystalline metal oxide is used as the negative electrode, and combined with 4V-class anode materials to build a practical battery, it may be claimed that the goal of EV storage device development has been achieved with this material, in consideration of weight of electrolyte solution and casing, in respect to both energy density and power density, as compared to other storage devices. The development goal has been set to increase by an order of magnitude or more in energy density (from 1 Wh/kg) and in power density (from 1 kW/kg), assuming that the electric double layer capacitor is applied to EV (Fig. 4).
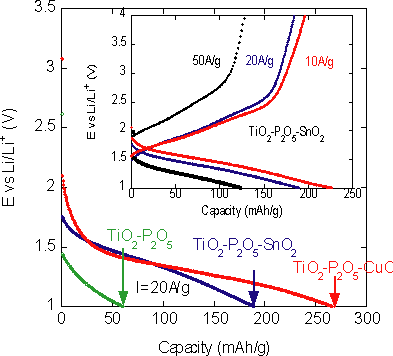 |
Fig. 3 The charge-discharge profiles of TiO2-P2O5 CGMN, TiO2-P2O5-SnO2 CGMN and TiO2-P2O5-CuO CGMN at 20A/g. The specific capacities 65mAh/g of TiO2-P2O5 CGMN at 20A/g is considerably improved to 195mAh/g and 270mAh/g for TiO2-P2O5-SnO2 and TiO2-P2O5-CuO, respectively. The inset shows that the specific capacities of TiO2-P2O5-SnO2 CGMN under current densities of 10A/g, 20A/g and 50A/g were 230mAh/g, 195mAh/g and 178mAh/g, respectively.
|
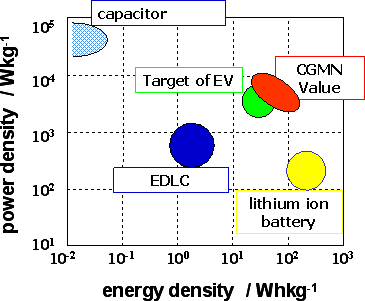 |
Fig. 4 A Regone plot shows the position of the power and energy density of CGMN as energy storage devise comparing with EDLC, Capacitor, a traditional rechargeable lithium battery, and the PNGV target. |